Other Concerted [n + m] Cycloadditions
- Concerted [2π + 2π] cycloaddition
- Nomenclature of cycloaddition reactions
- Thermal concerted [2π + 2π] cycloadditions
- Photochemical concerted [2π + 2π] cycloadditions
- Selection rules for [n + m] cycloadditions
1 Concerted [2π + 2π] cycloaddition
We can draw the thermal [2π + 2π] cycloaddition as shown below, but it is NOT generally useful. A quick analysis of the FMOs reveals why this is the case: the activation energy is high for both the forward and reverse processes (cyclobutanes do not give two moles of alkene on heating). The reaction is described as thermally forbidden.
Overall reaction:
![Simplified mechanistic scheme for a [2π + 2π] cycloaddition](images_PR/3003.png)
FMO interactions:
![HOMO-LUMO interactions in a [2π + 2π] cycloaddition](images_PR/3006.png)
For the FMO analysis we need the HOMO of one component and the LUMO of the other. In this case, when we try to overlap the reacting ends of the MOs it is impossible to have simultaneous bonding (in-phase) overlap for both forming σ bonds (Figure 3.2). No matter which HOMO–LUMO pair we use, there is one bonding interaction and one antibonding interaction; this kind of face-to-face thermal [2 + 2] cycloaddition cannot be a concerted process.
![Orbital interactions in a 'face-to-face' [2π + 2π] cycloaddition](images_PR/3009.png)
Despite this, many [2 + 2] cycloadditions are known. So how do they work? One alternative reaction type is the stepwise (i.e. non-concerted) mechanism, with the first bond being formed as above, and the second in a separate step. Concerted pericyclic [2 + 2] cycloadditions are also known, but before looking at them we should revise the terminology that is routinely used to define specific pericyclic reaction modes.
2 Nomenclature of cycloaddition reactions
In the previous section we saw that the thermal concerted [2 + 2] cycloaddition of one alkene to another, giving a cyclobutane, through face-to-face HOMO-LUMO mixing of the reacting π systems gives rise to one bonding and one antibonding interaction. The diagrams are adapted below to show an alternative way for the required bonding overlap to proceed, and introduce the terms suprafacial and antarafacial, which are used to define the precise way in which a π system participates in a pericyclic process.
![Suprafacial and antarafacial overlap in [2π + 2π] cycloadditions](images_PR/3012.png)
- When two new bonds are formed on the opposite sides of a π-bond or conjugated π-system, as in the CH2=CH2 component of the above right-hand diagram, the process taking place on that component is said to be antarafacial.
- When the new bonds are formed on the same side of a π-bond or conjugated π-system, as in the CHX=CHY component of the above right-hand diagram, the process taking place on that component is said to be suprafacial.
The letters a and s are used to represent the facial behaviour in cycloadditions. The thermally allowed version of the [2 + 2] cycloaddition is more fully referred to as a [π2s + π2a] process. Similarly the Diels-Alder reaction (Section 2.1) is a [π2s + π2s] cycloaddition, as both of the π components react suprafacially in that process. These descriptors of facial reaction mode are important, as they are required in defining the selection rules for pericyclic reactions.
3 Thermal concerted [2π + 2π] cycloadditions
The [π2s + π2a] is a thermally allowed concerted process, but the strict requirements of the mechanism are such that it is quite rare. One of the reacting alkene units must approach the other at right-angles to enable the required antarafacial bonding to be concerted. Only if we can persuade one of the alkenes to bond from opposite faces (antarafacially) will a concerted thermal [2 +2] cycloaddition be allowed. The following are the key points:
- A 'normal' face-to-face HOMO-LUMO approach would involve antibonding.
- If the alkenes were to approach at right angles, bonding could be concerted.
- The R (or H) groups attached to the alkene prevent the molecules from interacting in this way. If the interactions are reduced, the [π2s + π2a] reaction may be possible.
![Possible reaction geometry for thermal [2π + 2π] cycloaddition](images_PR/3015.png)
The steric demands of this type of transition structure are high, as the substituents on the alkenes tend to clash before the bonding overlap can fully develop. One circumstance in which this steric requirement is minimised is when one of the 2π components is a ketene rather than an alkene. Reactions of this kind, e.g. 027, can be highly effective.
![Thermal [2π + 2π] cycloaddition of a ketene to cyclopentadiene](images_PR/3018.png)
![Thermal [2π + 2π] cycloaddition of a ketene to cyclopentadiene](images_PR/3021.png)
4 Photochemical concerted [2π + 2π] cycloadditions
We saw earlier (reaction 002) that the concerted [2 + 2] cycloaddition of two alkenes can be induced with UV light (hν). Why is this [2 + 2] cycloaddition an allowed process?
![Photochemical [2π + 2π] addition of ethene to maleic anhydride](images_PR/3024.png)
The light used is not just any old light — its frequency must be such that it will be absorbed by one of the alkenes and promote one of the electrons from the HOMO to the LUMO. This generates the (first) electronically excited state of the alkene:
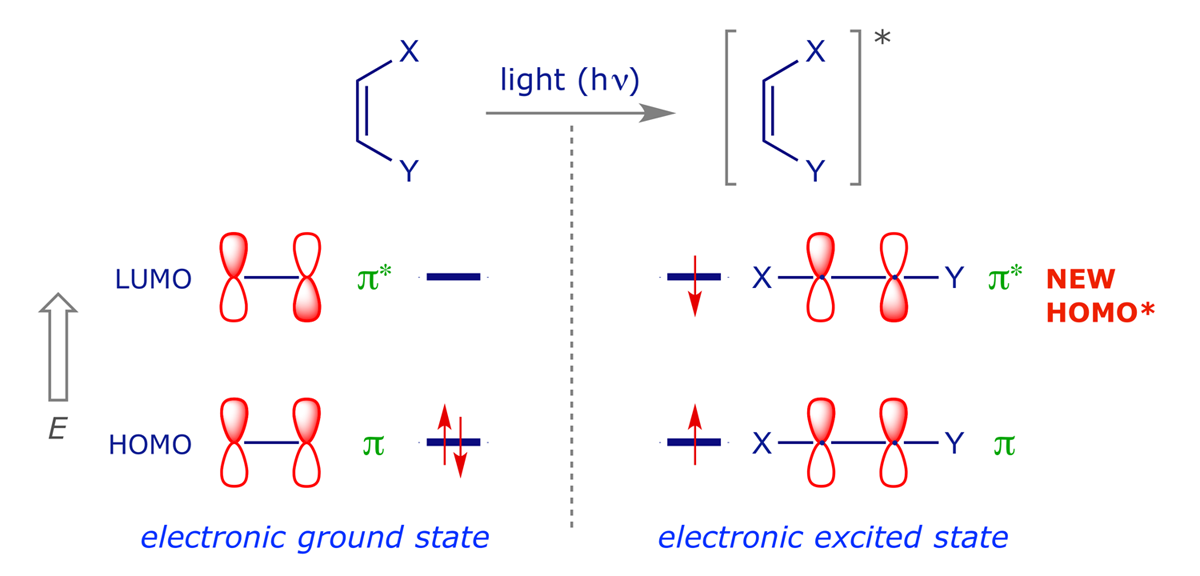
Most of the time the excited state simply decays back to the ground state (e.g. it might simply emit the light it absorbed), but occasionally it will encounter a ground-state alkene, and react to give the cyclobutane. The new orbital interaction diagram (below) shows that the key HOMO-LUMO interactions now both involve bonding overlaps, so the process is allowed.
![MO interaction diagram for [2 + 2] photoaddition of two alkenes](images_PR/3030.png)
For our FMO analysis we need to use the 'new' photochemical HOMO* (there is no LUMO for the excited alkene) and the LUMO of the other alkene.
![HOMO-LUMO interactions during the [2 + 2] photoaddition of two alkenes](images_PR/3033.png)
5 Selection rules for [n + m] cycloadditions
In the foregoing examples we have see the terminology of cycloadditions, some of which is general to all pericyclic processes, and we have seen the molecular orbital properties that give rise to the selection rules for 'allowed' and 'forbidden' reactions. The patterns which are emerging are summarised in the following table.
![Table showing selection rules for concerted [2 + 2] and [4 + 2] cycloaddition](images_PR/3036.png)
Suprafacial and antarafacial
In a process designated supra/antara, one component reacts suprafacially (both new bonds formed from the same face of the π system - either both above or both below) while the other reacts antarafacially (both new bonds formed from opposite faces of the π system - one above and one below). This is usually impossible because of geometric restrictions. In a process designated supra/supra, both components react suprafacially. This is usually easy and such reactions are the norm.
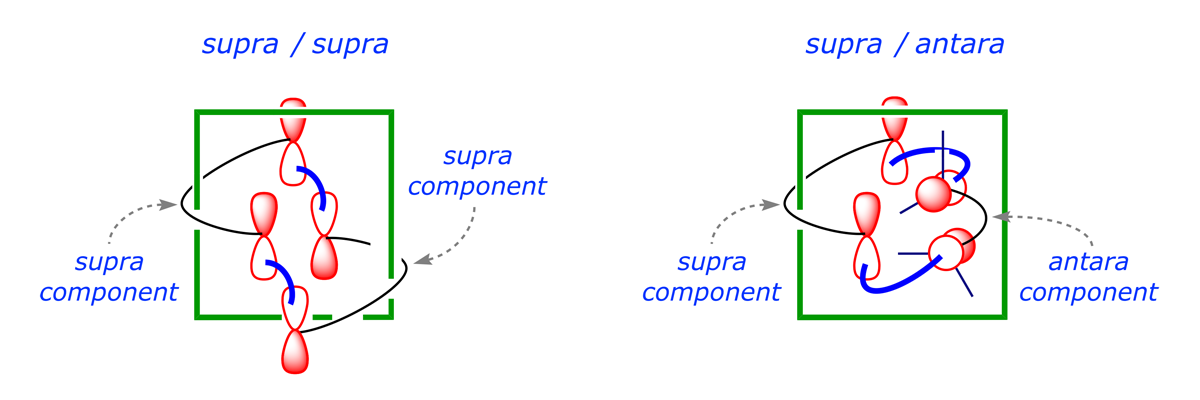
We can generalise these rules simply by considering the number of electrons in the transition structure (2 x the number of curved arrows). There is a pattern, and as we meet more examples of pericyclic reactions we will see it recurring, always for the same reason: the effects of orbital symmetry. This powerful idea allows us to predict which reactions will proceed easily (are classed as allowed and anticipate the stereochemistry of the product.
![Table showing the relationship between electron count and reaction mode for concerted [2 + 2] and [4 + 2] cycloaddition](images_PR/3042.png)
To make use of these selection rules, we carry out an electron count to identify the π system under scrutiny and note the phase pattern of its HOMO and LUMO — specifically at the termini that are directly involved in the pericyclic process. We have already constructed 1,3-butadiene (Introduction part 5.4); an extended set constructed in the same way is provided below.
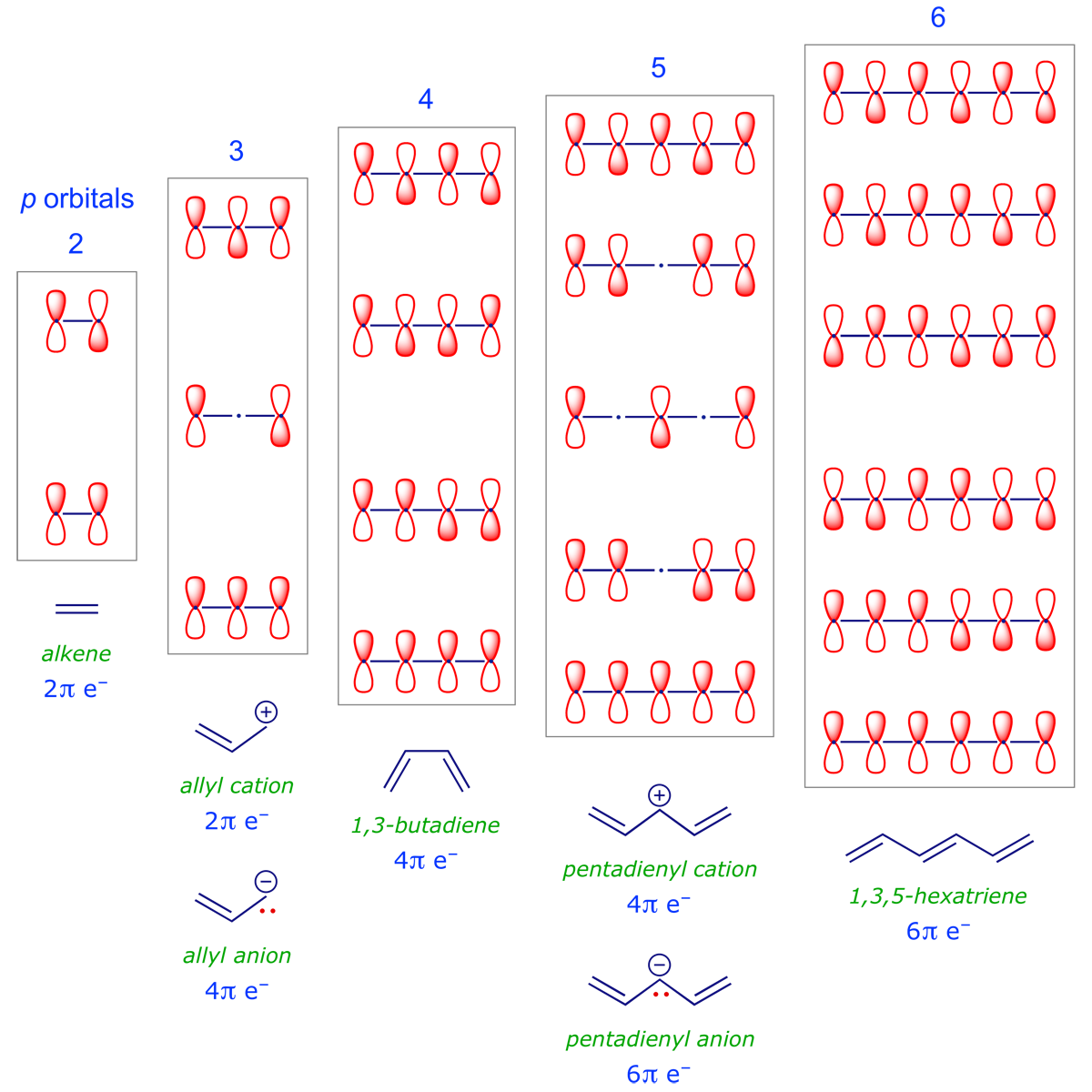
Recall that for a simple FMO analysis, all we need are the relative phases of the termini of the π system. The following patterns are evident:
- In any given system, the HOMO and LUMO have opposite symmetry. If the termini of the HOMO are in-phase, then the termini of the LUMO are out-of-phase.
- For adjacent systems differing by one π bond (two electrons), e.g. alkene and 1,3-butadiene, the respective HOMOs have opposite symmetry. The termini of one HOMO are in-phase and those of the adjacent HOMO are out-of-phase. The LUMOs of adjacent systems also follow this alternating pattern.
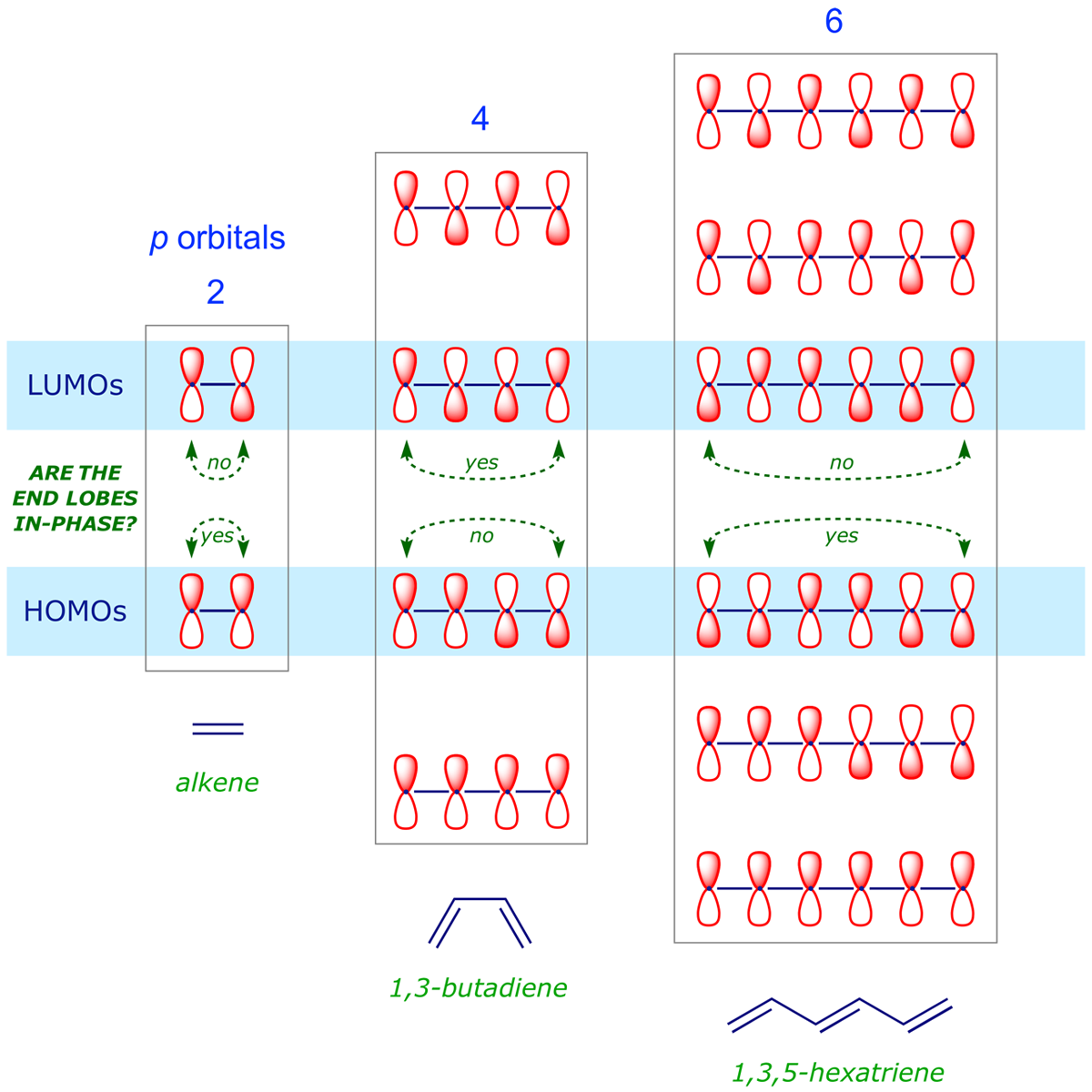
This alternating symmetry within π molecular orbitals is the basis of the above selection rules, i.e. the reaction mode switches between suprafacial and antarafacial as the number of electrons in a system switches between 4n and 4n + 2.
Fukui showed that the selection rules emerge intact from an analysis limited to the HOMOs and LUMOs of the reaction components. Woodward and Hoffmann provided a more general 'formula' for cycloaddition reactions based on the electron count:
- If (4n + 2) electrons are involved, allowed reactions are suprafacial about both components.
- If (4n) electrons are involved, allowed reactions are suprafacial about one component and antarafacial about the other.
The FMO approach to understanding cycloadditions, in particular the prediction of allowed or forbidden concerted bonding, thus involves the identification of the HOMOs and LUMOs of the reaction partners and the counting of the electrons involved in the cyclic transition structure.